Multistage Zeeman Deceleration
The ability to manipulate the translational motion of atoms and molecules using inhomogeneous magnetic fields offers new opportunities for precision atomic and molecular spectroscopy. To decelerate paramagnetic atoms and molecules, we have pioneered the technique of multistage Zeeman deceleration to generate slow beams of cold atoms or molecules [1]. In the experiments, the atoms or molecules are cooled during the expansion into vacuum from the high-pressure reservoir of a pulsed nozzle. The resulting supersonic atomic or molecular beams are characterized by a low temperature and a high velocity in the laboratory frame. A multistage Zeeman decelerator is an array of solenoids through which large currents are applied following carefully optimized temporal sequences. The current pulses are turned on and off so that dense packets of paramagnetic species are decelerated in a phase-stable manner, both in transverse and longitudinal directions [2]. The method can be used to generate slow beams of translationally cold molecules in a single magnetic sublevel of a single rovibrational level [3]. We have used multistage Zeeman deceleration to generate slow beams of H and D atoms [4], metastable Ne [5], O2 [3] and metastable He2 [6] and trap hydrogen and deuterium [7].
Such samples are ideally suited for performing high-resolution spectroscopic experiments because of the longer interaction times, the reduction of the Doppler effect and the elimination of spectral congestion. We currently focus on precision spectroscopic measurements of the Rydberg spectrum of He2, and on the determination, by Rydberg-series extrapolation techniques, of the rovibrational energy-level structure of He2+. The interest of precision measurements on few-electron molecules results from the fact that the properties of these molecules can be calculated extremely precisely using ab initio quantum-chemical methods which include the evaluation of relativistic and quantum-electrodynamics contributions to the molecular energies. The comparison of experimental and theoretical results in these systems thus enables rigorous tests of the theoretical predictions, potentially only limited by the uncertainties in fundamental constants.
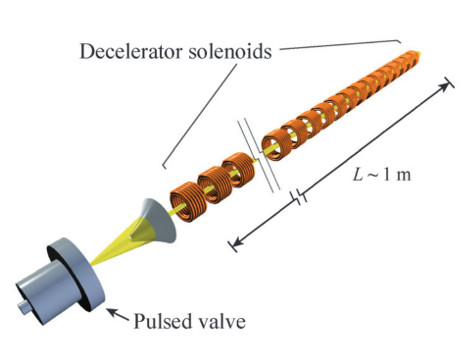
[1] "Multistage Zeeman deceleration of hydrogen atoms"
N. Vanhaecke, U. Meier, M. Andrist, B. H. Meier, and F. Merkt, Phys. Rev. A 75, 031402(R) (2007), doi: external page 10.1103/PhysRevA.75.031402
[2] "Phase stability in a multistage Zeeman decelerator"
A. W. Wiederkehr, S. D. Hogan, and F. Merkt, Phys. Rev. A 82, 043428 (2010), doi: external page 10.1103/PhysRevA.82.043428
[3] "Velocity-tunable slow beams of cold O2 in a single spin-rovibronic state with full angular-momentum orientation by multistage Zeeman deceleration"
A. W. Wiederkehr, H. Schmutz, M. Motsch, and F. Merkt, Mol. Phys. 110, 1807 (2012), doi: external page 10.1080/00268976.2012.681312
[4] "Slow beams of atomic hydrogen by multistage Zeeman deceleration"
S. D. Hogan, A. W. Wiederkehr, M. Andrist, H. Schmutz, and F. Merkt, J. Phys. B: At. Mol. Opt. Phys. 41, 081005 (2008), doi: external page 10.1088/0953-4075/41/8/081005
[5] "Multistage Zeeman deceleration of metastable neon"
A. W. Wiederkehr, M. Motsch, S. D. Hogan, M. Andrist, H. Schmutz, B. Lambillotte, J. A. Agner, and F. Merkt, J. Chem. Phys. 135, 214202 (2011), doi: external page 10.1063/1.3662141
[6] "Slow and velocity-tunable beams of metastable He2 by multistage Zeeman deceleration"
M. Motsch, P. Jansen, J. A. Agner, H. Schmutz, and F. Merkt, Phys. Rev. A 89, 043420 (2014), doi: external page 10.1103/PhysRevA.89.043420
[7] "Magnetic trapping of hydrogen after multistage Zeeman deceleration"
S. D. Hogan, A. W. Wiederkehr, H. Schmutz, and F. Merkt, Phys. Rev. Lett. 101, 143001 (2008), doi: external page 10.1103/PhysRevLett.101.143001
For further information, please contact us or learn more about our research by reading one of our Publications.